After hundreds of years of research and recent advances in imaging and biometry, we are close to understanding the mechanisms of accommodation and presbyopia. By building a computer-animated model, we can visualize multiple elements in the eye moving in synchrony, and with model-based reasoning, we can advance our understanding of the underlying mechanisms of accommodation.
There are countless pieces to solving this puzzle, including thousands of scientific studies and theories from the leading scientists of the 19th and 20th centuries. Hermann von Helmholtz’s lenticular theory of accommodation has been largely confirmed, but the role of extra-lenticular components in accommodation continues to be the subject of conflicting theories.
The movements of the ciliary body are now well documented, and the role of the ciliary body as the engine of accommodation is recognized. However, the distribution of forces via the zonular elements is not well understood. Nevertheless, recent landmark studies have defined the anatomy of the zonular architecture.1,2
Building the first model
A team of Europeans (Elke Lütjen-Drecoll and colleagues at the Institute of Anatomy in Erlangen, Germany), and Americans (Mary Ann Croft, Paul L. Kaufman, MD, and colleagues at the University of Wisconsin-Madison) have used electron microscopy, as well as video ultrasound biomicroscopy (UBM) and endoscopy, to define the complex separate elements of the zonule and to document the interconnected movements of the extralenticular tissues. Their monumental work makes it possible to now build a model and to understand and demonstrate the mechanism of accommodation.
Based on this new knowledge of the anatomy of the zonular architecture and aided by video UBM imaging of accommodation, in collaboration with a computer animator, my first model of accommodation was produced in 20113.
The concept of reciprocal zonular action was developed using model-based reasoning as follows: “During ciliary body contraction, the anterior zonules lose tension while the posterior zonules stretch and exert force on the posterior lens capsule playing a role in shaping the posterior lens thickness and curvature. During ciliary body relaxation, the posterior zonules lose tension as the lens flattens and is pulled back by the increasing tension of the anterior zonules.”3
Model 2.0
A second version of the model, “Computer-animated model of accommodation and presbyopia (CAMA), version 2.0,” has recently been presented.4 It is based on anatomy and biometry in accommodation and disaccommodation, endoscopy and video UBM and not on theory, and the findings are based on model-based cognitive reasoning.
It represents a biometrically accurate (structures within 200 microns) model of a 25-year-old eye with 8 diopters of accommodation, and helps us to understand and demonstrate the complex movements of accommodation.
Breaking down the elements (View video)
Figure 1 presents the composite model. The changes in shape and position of all anatomic elements are shown, demonstrating how the structures interconnect and function as a unit.
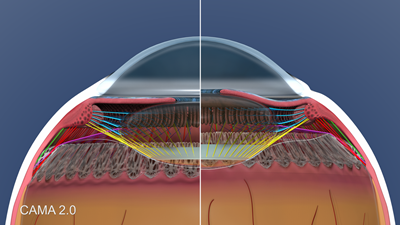
Figure 1. CAMA 2.0: Composite model showing structures in accommodation and disaccommodation.
It is very helpful to isolate the extralenticular elements as shown in figure 2. The anterior zonules (also known as zonules of Zinn) are shown in blue. There are three elements of vitreous zonules: the anterior vitreous zonule (shown in yellow), intermediate vitreous zonule (shown in red) and the posterior vitreous zonule (shown in off-white as the interconnecting network of fibers at the vitreous base). In addition, there is a pars plana zonule (shown in green), and the newly described PIZ-LE (posterior insertion zone to lens equator) zonule shown in purple. Also notice the representation of the anterior hyaloid membrane (white line) and Weiger’s ligament (cradle-shaped attachment of the posterior lens capsule and anterior hyaloid membrane) shown in grey.
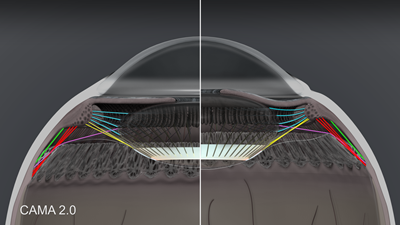
Figure 2. CAMA 2.0: Extralenticular structures in accommodation and disaccommodation.
New classification of zonular architecture
The present classification of zonules into anterior/posterior or lenticular/vitreous/pars plana is confusing. After analysis of the model, I’ve propose a new classification of zonular architecture based on structure and function.
Figure 3 demonstrates the first group of zonules as the anterior zonules whose function is to release tension on the lens during accommodation, allowing the intrinsic elasticity of the lens to “round-up” the lens and contributing to the increase in lens thickness and steepening of the anterior and posterior capsule. In disaccommodation, the anterior zonule increases tension and flattens the lens.
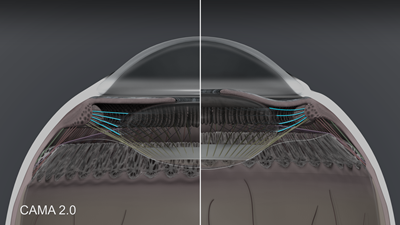
Figure 3. CAMA 2.0: Anterior zonules in accommodation and disaccommodation.
The second group of zonules is the crossing zonules shown in figure 4. Here the anterior vitreous zonule (yellow) is shown inserting into Weiger’s ligament, and the PIZ-LE zonule (purple) is shown anchoring the lens equator to the posterior insertion zone. The crossing zonules and Weiger’s ligament cradle shape and stabilize the posterior lens and vitreous. There needs to be something to prevent the lens from rattling around in the eye, like the eyes of a downhill skier that need to keep focusing while travelling quickly over bumpy terrain. Based on the model, the crossing fibers, combined with Weiger’s ligament, keep the lens in place while allowing the anterior and posterior zonules to provide reciprocal accommodation and disaccommodation of the lens.
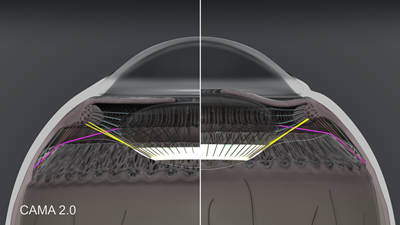
Figure 4. CAMA 2.0: Crossing zonules in accommodation and disaccommodation. Anterior vitreous zonule shown in yellow, PIZ-LE zonule in purple and Weiger’s ligament in white.
The third functional group is the posterior zonules shown in figure 5. These include the intermediate vitreous zonule (red), posterior vitreous zonule (off-white) and pars plana zonule (green). These are reciprocal tension fibers that follow ciliary muscle movement and are anchored in the elastic choroid.
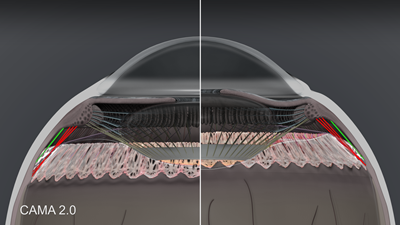
Figure 5. CAMA 2.0: Posterior zonules in accommodation and disaccommodation. Intermediate vitreous zonule shown in red, pars plana zonule in green, and posterior vitreous zonule in off-white.
Accommodation does not stop at the ora serrata. Movement of the ora has been demonstrated by tranillumination, UBM and endoscopy, and we are just beginning to appreciate the implications. Figure 6 demonstrates the elastic foundation in the choroid where energy for disaccommodation is stored.
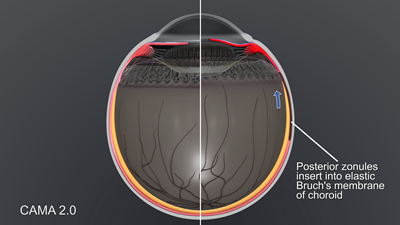
Figure 6. CAMA 2.0: Choroid and sclera in accommodation and disaccommodation.
Improving our understanding
By modeling the aqueous and vitreous spaces, we can add to our understanding of the hydrodynamics of accommodation.
Figure 7 demonstrates the accommodative movements. When the lens thickens and moves anteriorly during accommodation, the anterior chamber shallows centrally; however, the peripheral iris bends posteriorly, allowing the anterior chamber volume to equilibrate. The movement of aqueous in the posterior chamber, demonstrated by Croft, Kaufman and associates2 with endoscopy, shows that at the same time that aqueous is moving posteriorly through the circumlental space into the anterior hyaloid cleft, the anterior hyaloid in the region between Weiger’s ligament and the peripheral shoulder of the vitreous bows backwards. The hydraulic pressure in the posterior aqueous overcomes the pressure in the vitreous space. Within the vitreous space, the accommodative forward movement of the peripheral hyaloid membrane pulls forward much of the neighboring inner vitreous near and posterior to the region of the ora serrata. Axially, the posterior lens capsule moves posteriorly during accommodation resulting in posterior movement of the vitreous in this area behind Berger’s space.
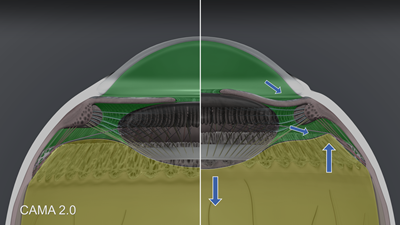
Figure 7. CAMA 2.0: Hydrodynamics of aqueous and vitreous. Aqueous in lime green and vitreous in yellow-green.
The hydrodynamics demonstrate that the vitreous space is passive, and internal movements of the vitreous body are related to movement of the surrounding structures of the uveal tract and lens. Instead of “vitreous pressure,” there may be pressure from the elastic stretch in the choroid transmitted to the vitreous.
Figure 8 presents a side-by-side comparison of a 25-year-old normal eye and a 50-year old presbyopic eye. The latter demonstrates thickening and stiffening of the lens and capsule, with steepening of the anterior capsule curvature. Also the lens equator moves forward with age and the insertion of the anterior zonules moves anteriorly on the lens capsule with age.
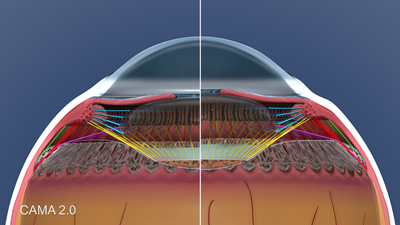
Figure 8. CAMA 2.0: 50 year old presbyopic eye in accommodation and disaccommodation.
Note that the anterior movement of the ora serrata is markedly reduced in presbyopia, which is related to the loss of elasticity in the ciliary body and choroid. It is evident that the aging of the extralenticular structures are major factors, in addition to the aging of the lens, in age-related loss of accommodative movement.
It is my hope that this second version of my model will help to identify new directions for research.
References
- Lutjen-Drecoll E, Kaufman PL, Wasielewski R, Ting-Li L, Croft MA. Morphology and accommodative function of the vitreous zonule in human and monkey eyes. Invest Ophthalmol Vis Sci. 2010;51(3):1554-1564.
- Croft MA, Nork TM, McDonald JP, Katz A, Lutjen-Drecoll E, Kaufman PL. Accommodative movements of the vitreous membrane, choroid, and sclera in young and presbyopic human and nonhuman primate eyes. Invest Ophthalmol Vis Sci. 2013;54:5049-5058.
- Goldberg DB. Computer-animated model of accommodation and theory of reciprocal zonular action. Clinical Ophthalmol. 2011(5):1559-1566.
- Goldberg DB. Computer-animated model of accommodation and presbyopia (CAMA 2.0). Paper presented at: ASCRS-ASOA Symposium and Congress; April 26, 2014; Boston. Best paper of session.